Deze pagina is momenteel niet beschikbaar in uw taal. U kunt met behulp van Google Translate een automatische vertaling bekijken. Wij zijn niet verantwoordelijk voor deze dienstverlening en het vertaalresultaat is niet door ons gecontroleerd.
Heeft u meer hulp nodig, neemt u dan contact met ons op.
What is Raman scattering
We explain the theory behind the Raman effect and how it was discovered by Prof. Sir C.V. Raman.
What is spectroscopy?
We use spectroscopy to measure the colours and relative intensity of light after it interacts with materials. Spectroscopy can tell us about the chemical composition, and physical or electronic structure of materials.
Light interacts with matter in different ways: transmitting through some materials, while reflecting or scattering off others. Both the material and the colour (wavelength) of the light affect this interaction.
Which parts of the visible spectrum enter our eyes determines which colours we perceive. For example, a substance might appear blue if it absorbs the red parts of the spectrum of light that fall upon it. Only the blue parts of the visible spectrum are reflected, or scattered, into our eyes.
The different fundamental light processes during material interaction.
Who discovered Raman spectroscopy?
The Raman scattering process is named after its discoverer, the famous Indian physicist Professor Sir Chandrasekhara Venkata Raman. Prof C.V. Raman and his student K.S. Krishnan showed that light undergoes a change in colour when it passes through a transparent material. It has changed colour and energy by interacting with molecular vibrations. This is the inelastic Raman scattering process. At the time, other scientists recognised the Raman effect as one of the most convincing proofs of quantum theory. Prof C.V. Raman was awarded the 1930 physics Nobel Prize in Physics for this great discovery.
Prof. Raman discovered the Raman effect in 1928. However, decades passed before advances in lasers, detectors, and computing led to the development of efficient Raman systems. Raman spectroscopy is now an essential tool in both laboratory and manufacturing environments.
eBook download: Raman spectroscopy explained
- What is the Raman effect?
- What is Raman spectroscopy?
- What can Raman imaging show you?
- Advantages of Raman spectroscopy
- Parts of a Raman spectrometer
- Photoluminescence explained
How do you detect the Raman effect?
You can measure the Raman effect with a Raman spectrometer. The first step is to illuminate your sample with a single colour of light, such as from a laser. If you were to shine blue light onto a material, you might expect to just see blue light reflected from it. Most of the light that scatters is unchanged in energy (Rayleigh scattered).
Only around 1 part in 10 million of the scattered light is Raman scattered. With a Raman spectrometer, you can detect the Raman-scattered light that has changed colour and shifted in frequency. It has changed frequency during the scattering process by interacting with molecular vibrations. Raman scattering occurs because photons (particles of light) exchange part of their energy with molecular vibrations in the material.
How does Raman spectroscopy measure vibrational modes?
Raman spectroscopy measures the energy difference between vibrational modes by analysing the scattered light. Scattering occurs when a photon polarises the electron cloud of a molecule and raises it to a “virtual” energy state. Raman scattering occurs if the photons change energy during the scattering process. This is because the excited molecule has relaxed to a higher or lower vibrational state than it had originally.
Raman scattering is inelastic because photons change energy by interacting with molecular vibrational energy levels. Raman scattering is called ‘Stokes' when the scattered light loses energy. Raman scattering is called ‘anti-Stokes' when the scattered light gains energy.
Stokes Raman scattering occurs when the molecule moves from the ground state to a virtual state, before dropping down to a higher energy vibrational state than it had originally. Anti-Stokes Raman scattering occurs when the molecule starts in a vibrationally excited state, moves to a virtual state, before finally relaxing to its ground state. We rarely use anti-Stokes Raman light as it is less intense than the Stokes. However, it does represent equivalent vibrational information of the molecule.
In contrast, Rayleigh scattering occurs when the molecule returns to its ground vibrational state. It releases a photon with the same energy as the incident photon. Therefore, Rayleigh scattered light has the same frequency and colour as the incident light. Rayleigh scattering is around 107 times more intense than Raman-scattered light. Modern spectrometers use highly efficient filters to remove the Rayleigh-scattered light. This makes it easy to detect Raman scattering.
A Jablonski diagram shows the energy changes during Rayleigh and Raman scattering. S0, S1, S2 are typical electronic energy levels, with higher energy vibrational levels.
The mechanism of Raman scattering is like infrared (IR) absorption spectroscopy, but different selection rules apply. For Raman scattering to occur, a change in molecular polarisability is required during vibration. You will see some vibrations in the Raman spectrum but not in the infrared spectrum, and vice-versa. For example, Raman spectroscopy can analyse the carbon bonds in diamond, unlike infrared absorption spectroscopy.
What does the Raman shift tell you?
The Raman shift is the energy difference between the incident laser light and the scattered light. This change in energy depends on the frequency of vibration of atoms in a molecule. By studying molecular vibrations, we can discover the chemical and structural composition of the material.
A large Raman shift or energy change tells us that the molecular vibrations are high frequency. This is due to light atoms held together by strong bonds. Conversely, a small Raman shift or energy change tells us that the molecular vibrations are low frequency. This is due to heavy atoms held together by weak bonds.
Parts of a Raman microscope
A typical Raman microscope starts with an optical microscope. This is coupled to an excitation laser, Rayleigh filters, a spectrometer and a detector. The Raman effect is very weak; only about 1 part in 10 million of the scattered light has a shifted colour. This is too weak to see with the naked eye, so we analyse the light with a highly sensitive Raman spectrometer.
Renishaw's inVia Raman microscope consists of:
1. single or multiple lasers from ultraviolet (UV 244 nm) to infrared (IR 1064 nm) that you can switch with a single click
2. high-quality objective lenses to focus the light onto the sample. These include highly confocal 100×, long working distance and immersion options
3. Rayleigh filters to separate the reflected and scattered light so that only the Raman light is collected by the spectrometer
4. motorised spectrometer lenses which are automatically optimised for each laser wavelength
5. master diffraction gratings with high dispersion and longevity to separate the Raman light into its constituent colours
6. a stable and sensitive thermoelectrically cooled (-70 ˚C) CCD detector
7. a PC for automated system control, data collection and analysis
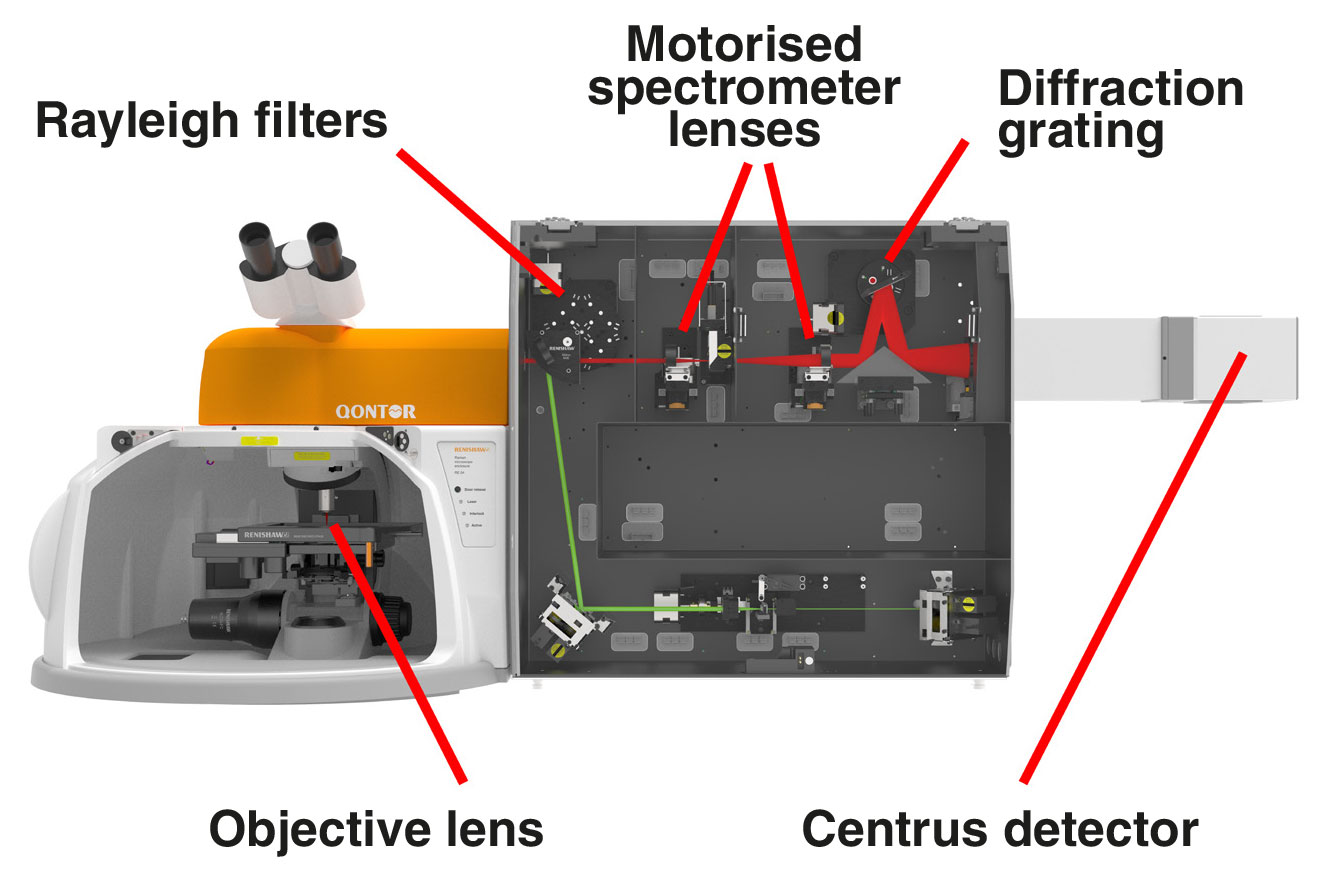
A typical optical layout for an inVia™ confocal Raman microscope
What is Raman spectroscopy?
Continue your exploration of Raman and photoluminescence (PL) spectroscopy. We answer your questions on Raman microscopy, fast Raman imaging, data analysis, fluorescence and complementary analytical techniques.
Raman spectroscopy explained