Why we use Raman spectroscopy
Learn about the advantages of Raman spectroscopy, and how it is ideal for combined and correlative microscopy. Raman microscopy complements other structural analysis techniques likes FTIR and XRD.
Raman spectroscopy is a versatile, non-destructive chemical analysis technique that usually requires no material preparation. We can combine Raman microscopy with other imaging and analytical techniques to gain multimodal information. Raman microscopy is complementary with Fourier-transform infrared spectroscopy (FTIR) and X-ray diffraction (XRD). We compare these analytical techniques.
Advantages of Raman spectroscopy
You can often save time on materials preparation and get straight to Raman analysis. Here are the main advantages:
You can get chemical composition and structure of materials with Raman analysis
Raman spectroscopy can detect different chemistries and structures. These include polymorphs that contain the same atoms but in different crystalline forms. You can also analyse mixtures and quantify their chemical composition.
Raman spectroscopy is widely applicable
All non-metals have active Raman spectra. This is why Raman spectroscopy is ideal for analysing unknown samples. Note that metal compounds do have Raman spectra. This is why Raman analysis can characterise metal oxides and help us to understand corrosion.
Raman analysis requires little sample preparation
If you can use an objective lens to light onto your sample, you can collect its Raman spectrum. You do not need any further sample preparation.
Raman microscopy enables label-free biochemical detection
You do not need any stains or dyes to differentiate between biological tissues and cells.
Raman analysis is non-contact and non-destructive
You can analyse your sample multiple times without changing it.
Raman microscopy can detect trace amounts
The best Raman microscopes can spatially resolve small amounts of material, below 1 micrometer in size. These include contaminants, nanodiamonds and monolayer graphene. Such systems use high numerical aperture lenses to efficiently illuminate and collect the Raman-scattered light from a tiny region of the sample.
Raman spectroscopy can analyse large samples and bulk chemicals too
We can use fibre-optic Raman probes for remote chemical analysis of immobile samples. Sometimes, we need remote in-situ Raman measurements (e.g. at a synchrotron beamline or in a chemical reactor).
Raman analysis is suitable for samples in water
You can analyse samples in aqueous solutions, such as colloidal suspensions, living cells or biochemical reaction mixtures. You do not have to extract or dry a wet sample. These processes take time and may also alter your samples chemistry or structure.
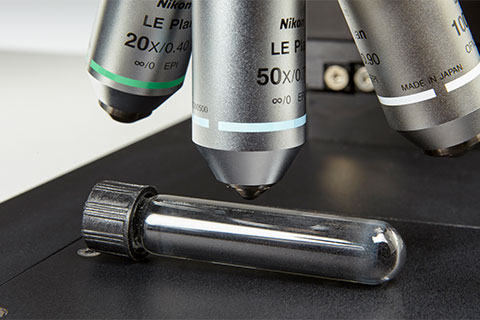
Correlative Raman microscopy
You can correlate the images from Raman microscopy with those from other forms of microscopy. The minimal sampling requirements and non-destructive nature of Raman spectroscopy makes this easy. We can easily integrate Renishaw's Raman systems with other techniques for co-localised measurements. These include:
- Scanning probe microscopy (SPM) / atomic force microscopy (AFM)
- Scanning electron microscopy (SEM) using the Renishaw inLuxTM SEM Raman interface
- Photocurrent imaging
- Photoluminescence (PL)
- Medium wavelength infrared thermography (MWIR)
- Nanoindentation
- Fluorescence-lifetime imaging microscopy (FLIM)
Together, these techniques can provide you with a complete understanding of your materials.
More information on combined Raman systems
Overlaid image showing a clear correlation between (A) the optical image, (B) the SEM image and (C) the Raman image. The Raman image shows the chemical composition of the polymer laminate as polycarbonate (red), rutile TiO2 (blue), poly[4,4'-methylenebis(phenyl isocyanate)- alt-1,4- butanediol/poly(butylene adipate) (green), copper phthalocyanine (cyan) and polyethylene terephthalate (yellow). Note the cyan layer which is not visible in the SEM or optical image, but is detected by Raman imaging.
eBook download: Raman spectroscopy explained
- What is the Raman effect?
- What is Raman spectroscopy?
- What can Raman imaging show you?
- Advantages of Raman spectroscopy
- Parts of a Raman spectrometer
- Photoluminescence explained
Raman microscopy compared with Fourier-transform infrared spectroscopy (FTIR)
Scientists often compare Raman spectroscopy to the more widely used Fourier-transform infrared spectroscopy (FTIR). Both are forms of vibrational spectroscopy. Raman and FTIR spectroscopy are complementary because their physical mechanisms differ.
FTIR measures how a sample absorbs light at distinct frequencies that correspond to the vibrational frequencies of molecular bonds. IR absorption requires a change in the dipole moment across a bond. As a result, FTIR spectroscopy is more sensitive to vibrations of heteronuclear functional groups with polar bonds, such as O-H stretching in water.
In contrast, Raman spectroscopy measures the inelastic scattering of light to probe molecular vibrations. For Raman scattering to occur, the incoming photon must induce a change in polarizability across a bond. Raman spectroscopy is more sensitive to vibrations of homonuclear molecular bonds and can differentiate C-C, C=C and C≡C bonds.
Both Raman and FTIR spectroscopy can be microscope based. Raman microscopy has some advantages over FTIR:
• Raman spectroscopy detects vibrational modes which are not IR-active.
• Raman microscopy can resolve smaller particles than microscope-based FTIR (micro-FTIR) because it often uses shorter wavelengths of light.
• Raman analysis can differentiate materials with higher chemical specificity due to narrower Raman bands.
• For FTIR analysis, samples may need to be in contact with an attenuated total reflectance (ATR) tip. Analysts must often prepare solid samples by grinding with Nujol mull or solvents. This is followed by compressing the sample mixture between KBr discs. Liquid samples may require NaCl plates.
• Quantitative Raman analysis may require a reference material does not require sample preparation with a fixed optical pathlength. Quantitative FTIR analysis is an absorption technique, so it relies on a consistent optical pathlength through the sample.
• Raman spectroscopy is suitable for depolarization studies of chiral molecules.
Raman microscopy compared with XRD
X-ray diffraction (XRD) is another non-destructive analytical method. You can use XRD to study physical properties such as phase composition, crystal structure and the orientation of solid or liquid samples. The wavelength of the X-rays is comparable to the distance between the atoms in a crystalline lattice. Constructive interference can thus occur between the incident X-rays and the crystalline sample. We can analyse the resulting XRD pattern to understand long-range order within the crystal structure.
Raman spectroscopy is complementary to XRD when for understanding the crystal structure of a material. However, Raman spectroscopy has some advantages over XRD:
• Raman spectroscopy can measure both crystalline and amorphous substances.
• Raman analysis can measure small amounts of sample down to single grains or particles.
• Raman spectroscopy does not need a vacuum chamber, humidity or temperature control.
Getting the best from your Raman system
While Raman spectroscopy has many advantages, it can present some challenges. Find out about the ways we can address some of the issues encountered during its use.
What is Raman spectroscopy?
Continue your exploration of Raman and photoluminescence (PL) spectroscopy. We answer your questions on Raman microscopy, fast Raman imaging, data analysis, fluorescence and complementary analytical techniques.
Raman spectroscopy explained